by Tryphonas Petrou, Senior Mechanical Engineer, Safety at Sea Ltd
[Originally published in IMarEST Marine Professional magazine, September 2023]
Goal-based Safe Return to Port (SRTP) regulations hinge on the concept that the ship itself is its own best lifeboat. They were introduced to increase the safety of passenger ships in case of fire and flooding casualties and to reduce the likelihood of evacuation.
The concepts of redundancy through separation and duplication, and the protection of equipment, are well-established strategies applied from the early design stages to ensure SRTP requirements are met. Taking power generation and propulsion as an example, these systems are generally duplicated and separated, effectively splitting the vessel into two zones, which then dictates the placement of these systems and necessary auxiliaries. This is a decision made at the concept design phase and it affects the spatial arrangement, the passive and active fire protection as well as the size and location of various tanks.
In general, such arrangements work well, however, there are instances where these established strategies are further challenged due to factors such as SRTP fuel requirements exceeding the capacity of the service tanks. In this instance, the fuel-transfer system may be required to transfer fuel from areas outside the dedicated propulsion areas, and therefore cross the boundaries of the previously described separation – and potentially through spaces affected by the fire or flood casualty.
For this article, we used our proprietary digital solution Systema™ to analyse a case study, troubleshooting at the concept design phase and reducing the risk of issues as the design evolves.
Concept stage considerations
During concept design, it is not possible to design systems in detail, but it is important to consider system arrangement in terms of main components and pipe routings, and the spaces they occupy. These simplified arrangements can be modelled in Systema™ and assessed at the early stage against the SRTP requirements and own design philosophy.
For this example, a simplified fuel-transfer system was considered consisting of two fuel-transfer pumps (one in the pump/fueltransfer room, and the other in the forward engine room), which take fuel from one of two marine gasoil tanks located just forward of midships, to supply dedicated service tanks in each engine room. For the purposes of the example, the power supplies to the pumps were not considered, but this could be included if the information was available. The same applied for the venting lines of the storage tanks. For the tank air vents of each engine room, these are routed via their respective casing.
The fuel-transfer system is arranged in four watertight compartments extending over two decks as indicated in Figure 1. The two engine rooms (indicated in green and grey) each have their own service and overflow tanks, highlighted by purple and orange respectively. Each storage tank has enough capacity to replenish both service tanks for the whole duration of an SRTP voyage. Therefore, as long as one engine room is available, and fuel can be transferred to its service tank, the SRTP requirements will be met.
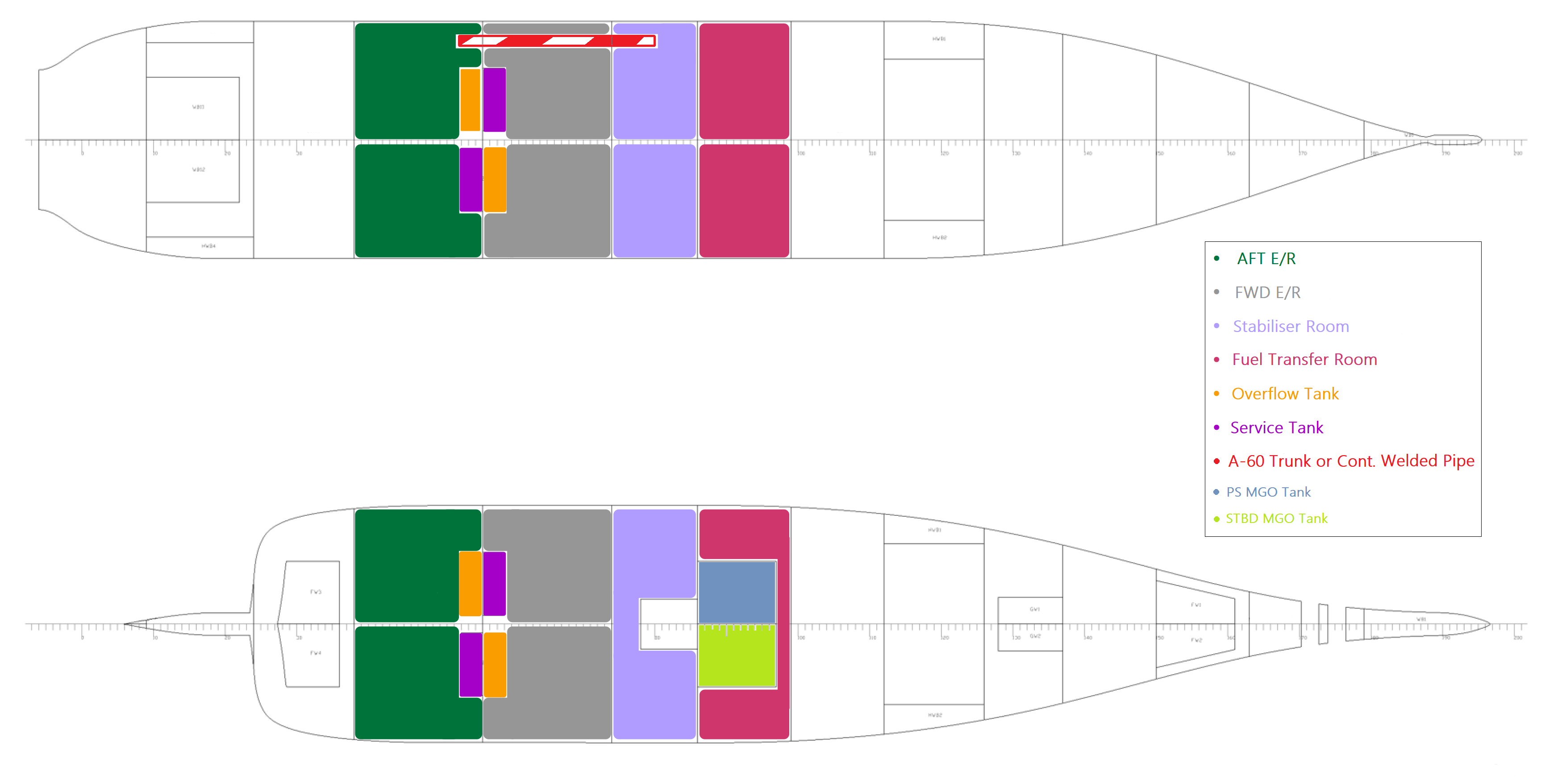
According to assumptions of an SRTP assessment, all the items contained in an affected compartment are lost, with some exceptions related to the protection of equipment and contents of piping. Figure 2 visualises the impact of a casualty on a system for a fire in the forward engine room.
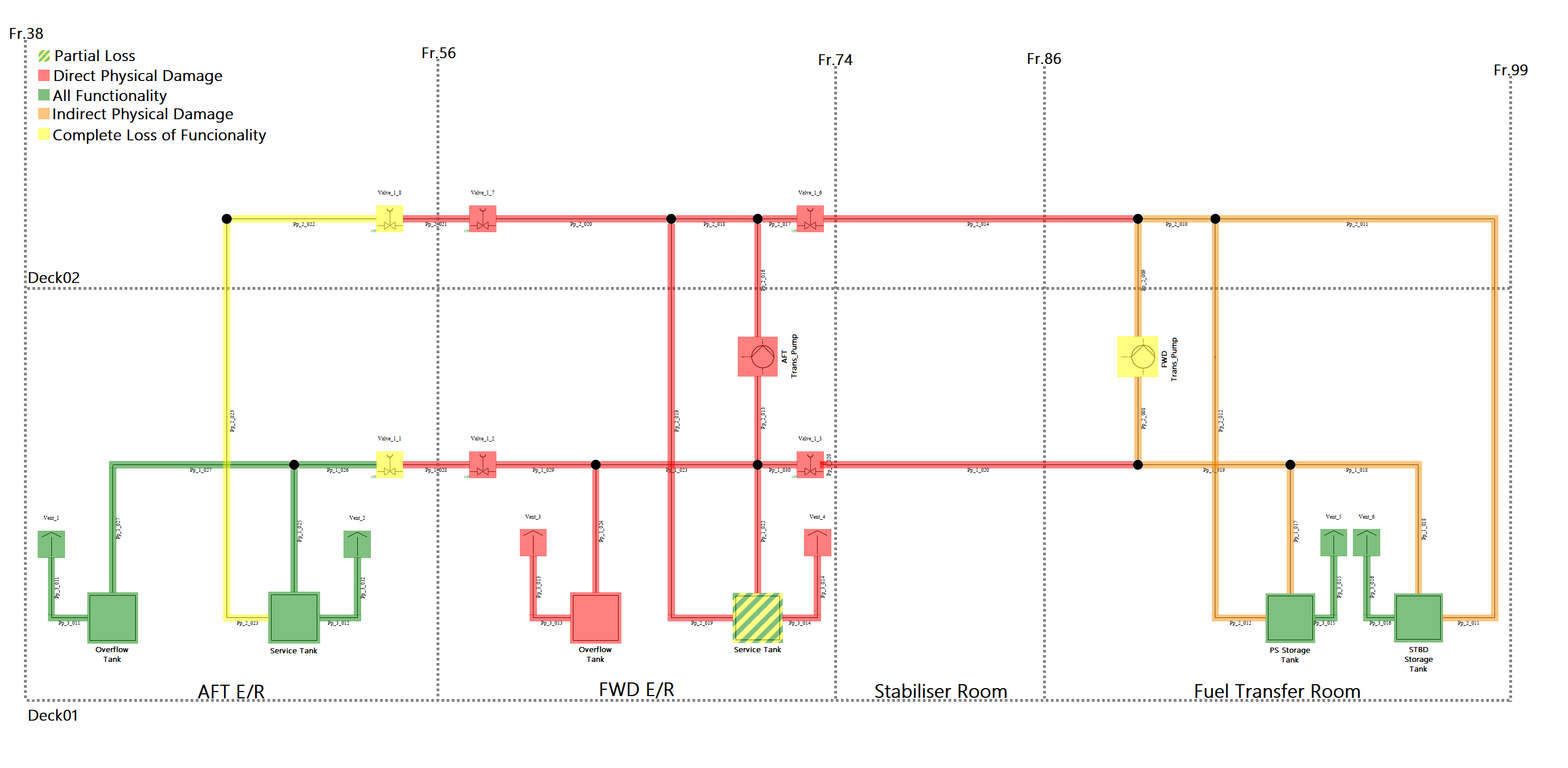
Visual identification
Systema™ can visually identify failure causes or make use of the inbuilt root cause analysis, which can automatically identify and report the causes of failure. In this case, this root cause analysis identified the fuel lines passing through the forward engine room and stabiliser room, which are required to supply the aft engine room, which furthermore affects the forward fuel-transfer pump’s operation due to the depressurisation. This is also caused partly by the current location of the containment valves, which are installed in the aft and forward engine rooms only.
Through visual or root cause analysis it is evident that improvements to containment are required, but this is only part of the issue as the fuel lines pass through the casualty space, and so this must also be overcome. As far as the containment concerns, adding additional valves in the fuel-transfer room protects it from casual ties aft and in terms of restoration, there are two possible solutions: install an A60 trunk between frames 86 and 56 with a fuel pipe routed within or the use of a continuous welded pipe and spool pieces between the aft engine room and the stabiliser room. Both solutions incorporate normally closed valves at both ends of these arrangements, which should open for fuel transfer.
Another issue is the loss of fueltransfer room, which causes a loss of supply to both engine rooms. By adding additional containment valves aft of the bulkhead at frame 86 and rerouting the suction and fill-up lines of the PS storage tank to the stabiliser room, it ensures that marine gasoil will still be transferred even though the fueltransfer room is lost.
In the case study, these changes were applied and the analysis was rerun. The new results are shown on Figure 3, showing the supply of fuel to the aft engine room can be protected and restored (see the blue highlighting).
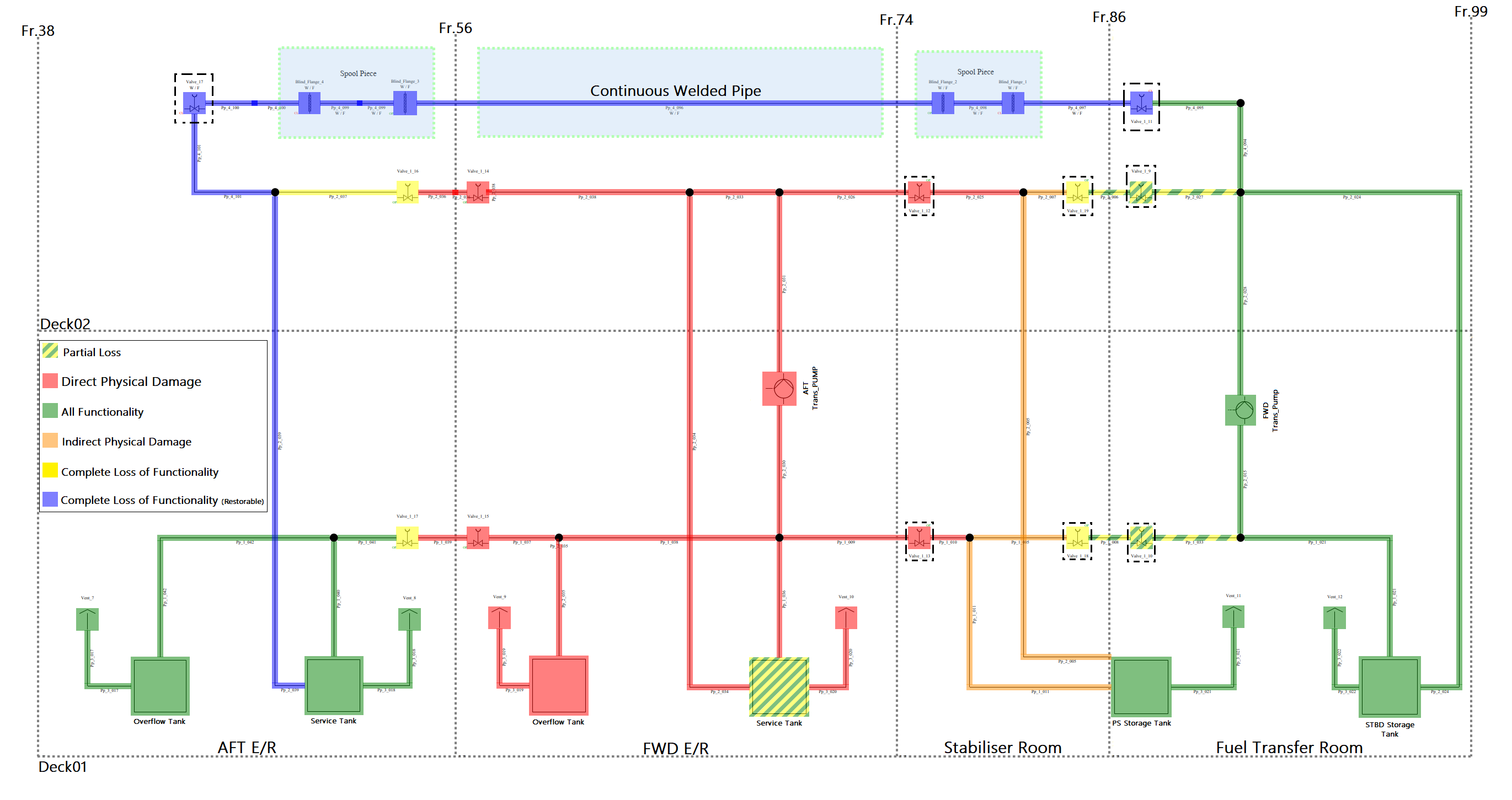
The benefit of using a digital tool for analysis and troubleshooting is its ability to highlight hidden design flaws as early as the concept design stage and enable the stakeholders to come up with alternative solutions. Corrections can be implemented and tested using the software, reducing the risk at the later design phases, where cost and time implications are critical.
For more information about this example refer to the following link: https://safetyatsea.uk/systema-for-concept-design/